LiFePO4 (Lithium Iron Phosphate) batteries are transforming energy storage systems with their industry-leading 4,000+ cycle lifespan, exceptional safety profile, and reliable performance across temperatures from -20°C to +60°C. For system integrators, solar installers, and battery owners, proper maintenance isn’t just recommended—it’s essential for maximizing your investment. Each improper charging cycle or storage mistake can reduce capacity and performance, potentially costing thousands in premature replacements. This data-driven maintenance guide combines technical expertise with practical steps to extend battery life by up to 30%, maintain peak efficiency, and avoid the most common maintenance mistakes that compromise LiFePO4 performance in real-world applications.
LiFePO4 Battery Fundamentals
LiFePO4 batteries represent a significant advancement in lithium battery technology, offering unique advantages that distinguish them from other energy storage solutions. Understanding these fundamental characteristics provides the foundation for proper maintenance and care.
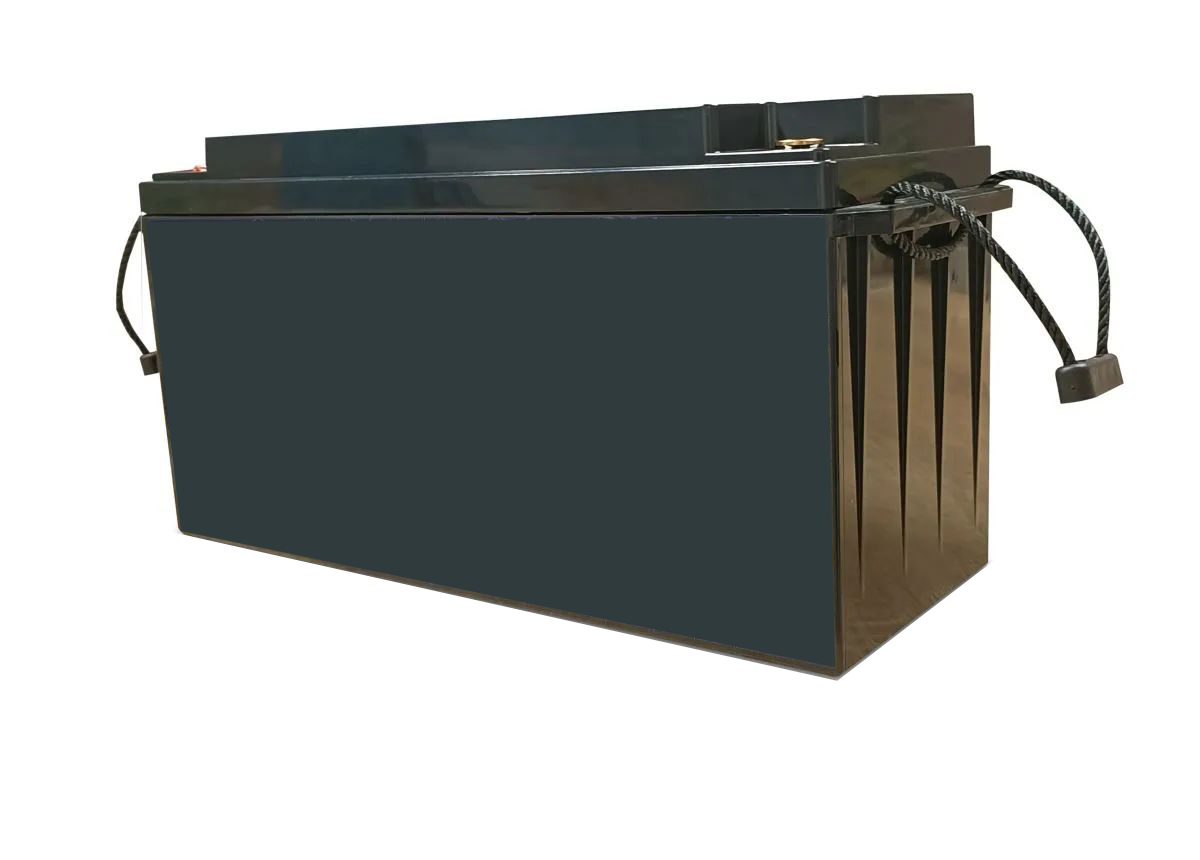
Chemistry and Structural Advantages
LiFePO4 batteries utilize lithium iron phosphate as the cathode material, creating exceptional thermal and chemical stability. The P-O bond in the (PO4)3- ion is stronger than the Co-O bond found in traditional lithium cobalt oxide batteries, allowing oxygen atoms to release more slowly when subjected to abuse conditions. This inherent stability significantly reduces thermal runaway risk, making these batteries substantially safer for diverse applications.
The structural integrity of LiFePO4 batteries remains consistent throughout charge cycles. Unlike LiCoO2 cells that undergo non-linear expansion affecting structural integrity during lithium migration, the fully lithiated and unlithiated states of LiFePO4 maintain similar structures. This stability ensures the battery retains its physical integrity throughout its operational life.
Performance Characteristics
LiFePO4 batteries deliver impressive performance metrics that make them ideal for demanding applications. They feature a power-to-weight ratio of 250-670 W/kg and maintain a nominal cell voltage of 3.2-3.3V. Their discharge and charging efficiency reaches up to 90%, significantly outperforming lead-acid alternatives that typically achieve only 80% efficiency.
These batteries perform exceptionally across broad temperature ranges (-20°C to +60°C), ensuring reliable operation in various environments. Their self-discharge rate remains remarkably low at approximately 2% monthly, compared to lead-acid batteries that may lose around 30% of their charge monthly when idle.
One of the most compelling advantages of LiFePO4 technology is its extraordinary longevity. While traditional batteries might offer 300-500 charge cycles, quality LiFePO4 batteries routinely deliver over 4,000 cycles, with premium variants exceeding 6,000 cycles. This translates to approximately 10 years of daily use, representing exceptional value for those investing in this technology.
Safety Profile
The safety profile of LiFePO4 batteries stands as their most compelling advantage. They demonstrate intrinsic thermal and chemical stability that significantly reduces fire and explosion risks, even under adverse conditions. Unlike cobalt-based lithium batteries that can become unstable when damaged or overcharged, LiFePO4 chemistry resists thermal runaway even when subjected to abuse.
During thermal testing, LiFePO4 batteries can withstand temperatures between 350°C-500°C before experiencing thermal issues—far higher than most other lithium chemistries. No lithium remains in the cathode of a fully charged LFP cell, whereas approximately 50% remains in a LiCoO2 cell, further enhancing their safety profile.
The stability of LiFePO4 chemistry extends to environmental safety considerations. These batteries contain no rare earth metals, toxic materials, or caustic substances, minimizing environmental impact during manufacturing, use, and eventual disposal.
Optimal Charging Practices for LiFePO4 Batteries
Proper charging practices significantly impact LiFePO4 battery performance and longevity. Following manufacturer-recommended protocols ensures maximum efficiency while preventing premature degradation.
Charger Selection and Specifications
Selecting an appropriate charger represents perhaps the most critical maintenance decision for LiFePO4 battery owners. Using chargers specifically designed for LiFePO4 chemistry is essential, as these provide the correct voltage and current profiles aligned with the battery’s specific requirements.
Charging voltage specifications vary based on battery configuration:
- 12V LiFePO4 batteries: 14.4V to 14.6V charging voltage
- 24V LiFePO4 batteries: 28.8V to 29.2V charging voltage
- 36V LiFePO4 batteries: 43.2V to 43.8V charging voltage
- 48V LiFePO4 batteries: 57.6V to 58.4V charging voltage
Modern LiFePO4 chargers typically feature automatic voltage detection and adjustment capabilities, ensuring the correct voltage during each charging phase. This precision prevents potentially damaging overcharging while maximizing capacity utilization.
Charging Profiles for Maximum Lifespan
The optimal charging profile for LiFePO4 batteries follows a multi-stage approach that balances charging speed with battery preservation. The most effective charging sequence includes:
A constant current (CC) phase where the charger delivers steady current until the battery reaches approximately 90% capacity. This is followed by a constant voltage (CV) phase where voltage remains fixed while current gradually decreases until full charge is achieved. Some advanced chargers include a final float or maintenance phase that applies a lower voltage to maintain charge without stressing the battery.
LiFePO4 batteries benefit significantly from opportunity charging—recharging whenever convenient rather than waiting for deep discharge. Shallow cycle charging generally extends battery life compared to frequent deep discharge cycles, though LiFePO4 chemistry can tolerate occasional deep discharges without significant degradation.
For optimal longevity, charge LiFePO4 batteries before they reach 20% state of charge (80% depth of discharge). While these batteries handle deep discharges better than most alternatives, maintaining some charge helps the battery management system function properly.
Temperature Considerations During Charging
Temperature significantly impacts charging efficiency and safety. The ideal charging temperature range spans 10°C (50°F) to 40°C (104°F), with optimal performance between 15°C and 30°C (59°F to 86°F).
Charging LiFePO4 batteries below freezing (0°C/32°F) must be avoided as this can cause lithium plating on the anode, permanently damaging capacity and performance. Advanced battery management systems automatically prevent charging at low temperatures to protect against this damage mechanism.
At high temperatures (above 45°C/113°F), charging efficiency decreases while accelerating component degradation. Quality management systems include temperature sensors that adjust charging parameters based on battery temperature or halt charging entirely when temperatures exceed safe thresholds.
During extreme weather conditions, allow batteries to acclimate to moderate temperatures before initiating charging. This prevents thermal shock while ensuring optimal charging efficiency and preserving battery lifespan.
Common Charging Mistakes to Avoid
Several charging practices significantly reduce LiFePO4 battery performance and longevity:
Using inappropriate chargers designed for lead-acid or other lithium chemistries represents the most damaging mistake. These chargers deliver incorrect voltage profiles that can permanently harm LiFePO4 batteries. Always use chargers specifically designated for LiFePO4 chemistry to prevent costly damage.
Overcharging, while less immediately damaging than with other lithium chemistries, still degrades LiFePO4 batteries over time. Quality chargers with proper termination parameters prevent this issue by automatically stopping the charging process when appropriate voltage thresholds are reached.
A common mistake for recreational vehicle owners involves using built-in chargers designed for lead-acid batteries to charge LiFePO4 batteries. These chargers typically provide inappropriate charging profiles that may damage LiFePO4 batteries over time.
Rapid charging at high currents, particularly for batteries not designed for high C-rates, generates excessive heat that stresses battery components. Unless specifically rated for fast charging, use moderate charging rates to maximize battery lifespan and preserve capacity.
Storage and Maintenance Best Practices
Proper storage and regular maintenance ensure LiFePO4 batteries maintain peak performance throughout their operational life. Following these guidelines prevents capacity loss while extending usable lifespan.
Proper Storage Conditions
The storage environment significantly impacts LiFePO4 battery health when not in use. Maintain dry, well-ventilated conditions with temperatures between 10°C to 25°C (50°F to 77°F). Extreme temperatures accelerate capacity loss and aging processes even during storage periods.
State of charge (SOC) during storage represents a critical factor in preserving battery health. Store LiFePO4 batteries at partial charge levels between 40% and 60% capacity. This charge state minimizes internal degradation while ensuring the battery management system maintains sufficient power for protective functions.
Different storage durations require specific considerations:
- For one month storage: Temperatures up to 30°C (86°F) generally remain acceptable
- For 3-6 month storage: Maintain temperatures between 10°C-20°C (50°F-68°F)
- For extended storage beyond 6 months: Keep temperatures near 15°C (59°F) with periodic charge maintenance
Before placing batteries in storage, disconnect all loads and peripheral devices. Even when powered off, BMS systems, inverters, or monitoring equipment draw small currents that gradually deplete the battery during storage periods.
Recommended Maintenance Schedule
Despite their “maintenance-free” reputation compared to lead-acid alternatives, LiFePO4 batteries benefit from regular attention to ensure optimal performance:
Monthly monitoring includes checking battery voltage and inspecting physical condition, ensuring connections remain secure and clean. Batteries with built-in monitoring systems should have diagnostic data reviewed for early warning indicators.
Quarterly maintenance for batteries in storage or experiencing infrequent use involves a shallow discharge (approximately 20%) followed by full recharge. This process prevents capacity loss while maintaining proper cell balance. Batteries with Bluetooth functionality require charging every three months to prevent BMS disconnection that could potentially disable the battery.
Semi-annual inspection should include thorough evaluation of the entire battery system, including capacity testing when possible, connection inspection for corrosion or damage, and exterior cleaning to prevent dust accumulation that impedes heat dissipation.
Annual performance assessment involves controlled capacity testing to evaluate whether the battery maintains its rated capacity. This process requires full discharge and recharge under controlled conditions while measuring actual energy delivery against rated specifications.
Voltage Monitoring and Cell Balancing
Voltage monitoring represents an essential maintenance practice, particularly for multi-cell LiFePO4 battery packs. Individual cells may develop voltage divergence over time, creating imbalances that reduce usable capacity and potentially lead to premature failure if left unaddressed.
The normal voltage range for a single LiFePO4 cell includes:
- Fully charged: 3.6-3.65V
- Nominal operating voltage: 3.2-3.3V
- Discharged state: 2.5-2.8V
Batteries stored for extended periods should have voltage checked every 3-6 months. If voltage falls below 3.0V per cell, recharge immediately to prevent deep discharge damage.
Cell balancing remains critical for multi-cell battery packs. Most commercial LiFePO4 batteries include battery management systems (BMS) that automatically perform cell balancing functions. The BMS ensures all cells maintain similar voltage levels by selectively redirecting current around cells that reach full charge before others.
For batteries without integrated BMS or systems with multiple connected battery packs, periodic manual balancing may be necessary. This typically involves a full charge cycle using balance chargers that monitor and control individual cell charging.
Troubleshooting Common Issues
Despite their reliability, LiFePO4 batteries occasionally exhibit issues requiring troubleshooting:
Reduced capacity often represents the most common concern. When a battery delivers noticeably less runtime than expected, check for parasitic loads draining the battery during idle periods. Verify the charging system functions correctly and delivers appropriate voltage. Extreme temperatures, particularly high heat, temporarily reduce available capacity. Significant capacity loss within the warranty period may indicate manufacturing defects requiring professional evaluation.
BMS lockout occurs when the battery management system detects potentially harmful conditions and disconnects to prevent damage. Common triggers include over-discharge, overcharging, short circuits, or operation outside safe temperature ranges. Resolving this typically requires connecting to an appropriate charger to “wake up” the BMS. Extremely low voltage batteries may require specialized recovery chargers.
Charging problems where batteries won’t accept charge or charge very slowly stem from several potential causes. The charger may be undersized for the battery’s capacity, connections may be loose or corroded, extreme temperatures may limit charging rates, or the BMS may restrict charging due to detected abnormalities. Check all connections, ensure appropriate environmental conditions, and verify charger compatibility with battery specifications.
For physically damaged or swollen batteries, immediately discontinue use and follow proper disposal procedures. Physical damage compromises safety features and may lead to thermal events or electrolyte leakage requiring specialist handling.
LiFePO4 in Solar and Backup Power Systems
LiFePO4 batteries excel in renewable energy applications, offering efficiency and longevity advantages that significantly enhance system performance and economics.
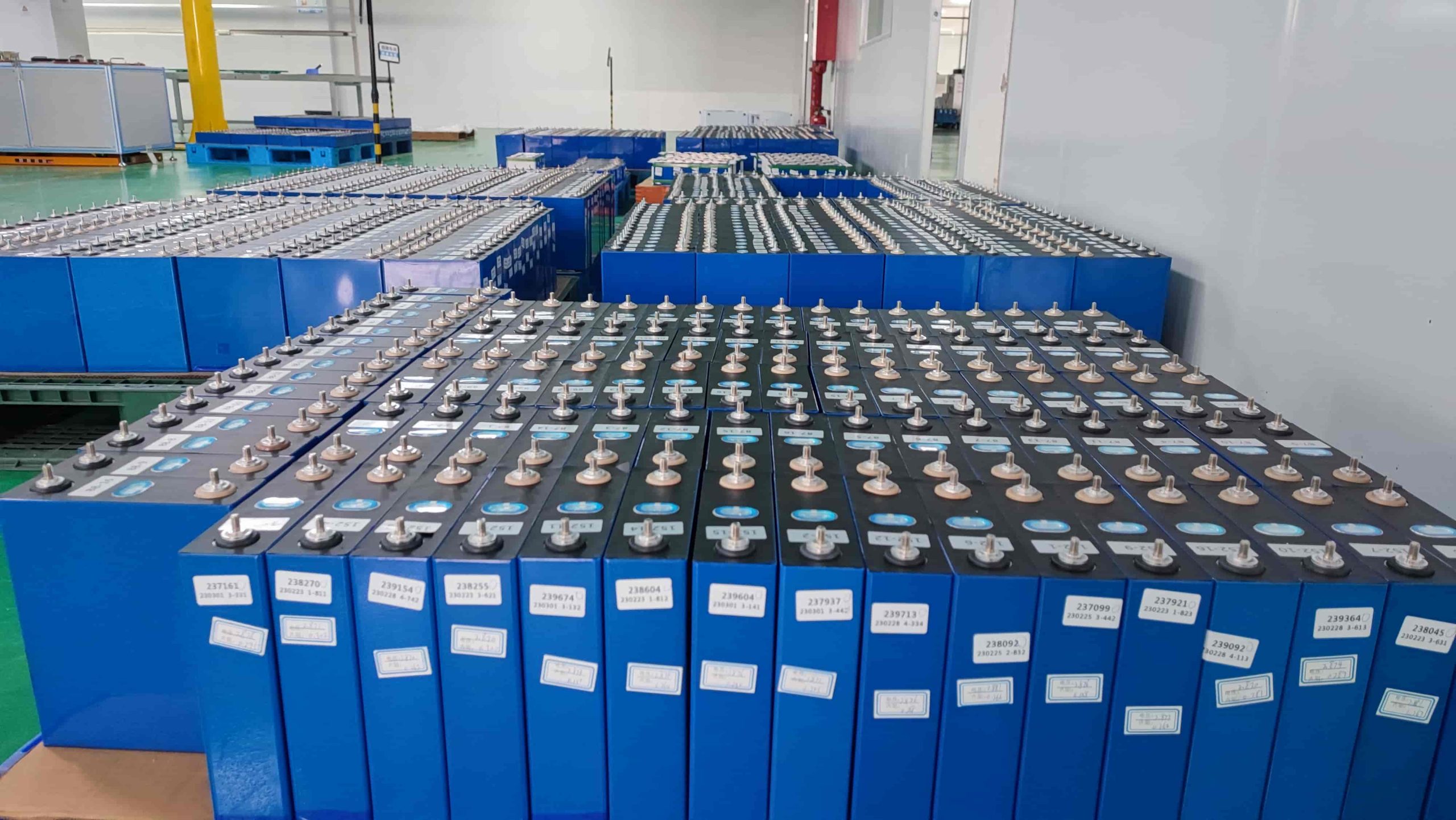
Integration with Renewable Energy Systems
LiFePO4 batteries integrate exceptionally well with solar power systems, where their high efficiency maximizes energy capture. Their ability to store excess solar production during daylight hours for evening use creates self-sufficient energy solutions that minimize grid dependence.
In solar applications, LiFePO4 batteries function within systems including solar panels, charge controllers, inverters, and monitoring equipment. Their high charge and discharge efficiency (approximately 90%) ensures minimal energy loss compared to lead-acid alternatives that typically achieve only 80% efficiency.
The consistent voltage delivery throughout discharge cycles ensures stable power to connected appliances and systems, particularly important for sensitive electronics that require uninterrupted clean power.
LiFePO4 batteries perform equally well in wind energy storage applications, where they manage intermittent generation patterns effectively. By storing energy produced during windy periods, these batteries provide continuous power regardless of momentary wind conditions.
Hybrid energy systems combining multiple renewable sources (solar, wind) with grid power benefit tremendously from LiFePO4 storage. These complex systems require energy solutions that handle frequent cycling, varying charge rates, and inconsistent usage patterns—all conditions where LiFePO4 chemistry excels.
Sizing LiFePO4 Banks for Applications
Properly sizing LiFePO4 battery banks ensures they meet energy demands while maximizing lifespan. The sizing process requires calculating daily energy consumption, determining desired autonomy days, and considering depth of discharge limitations.
To size a battery bank for solar applications, first calculate total daily energy consumption in kilowatt-hours (kWh), including all devices running on the system. For example, a household consuming 10kWh daily would use this figure as its baseline requirement.
Next, determine desired autonomy days—how long the system should operate without energy production. For systems designed with two-day autonomy during cloudy weather, multiply daily consumption by two to determine total energy storage requirements.
Consider depth of discharge (DoD) limitations when calculating total capacity requirements. LiFePO4 batteries typically allow 80% DoD without lifespan impact. To account for this limitation, divide total energy needs by 0.8 to determine required capacity.
For different applications, specific considerations apply:
- Residential backup systems focus on critical loads and typical outage duration
- Off-grid installations calculate seasonal variations in both production and consumption
- RV/Marine applications balance available space and weight constraints with energy requirements
- Commercial systems analyze peak demand periods and load-shifting opportunities
Comparative Advantages Over Lead-Acid
In standby and backup power applications, LiFePO4 batteries offer remarkable advantages over traditional lead-acid alternatives:
Cycle life represents the most dramatic difference between technologies. While lead-acid batteries typically provide 300-500 cycles at 50% DoD, LiFePO4 batteries routinely deliver 4,000+ cycles at 80% DoD. This translates to lifespan approximately 8-10 times longer, significantly reducing replacement frequency and associated costs.
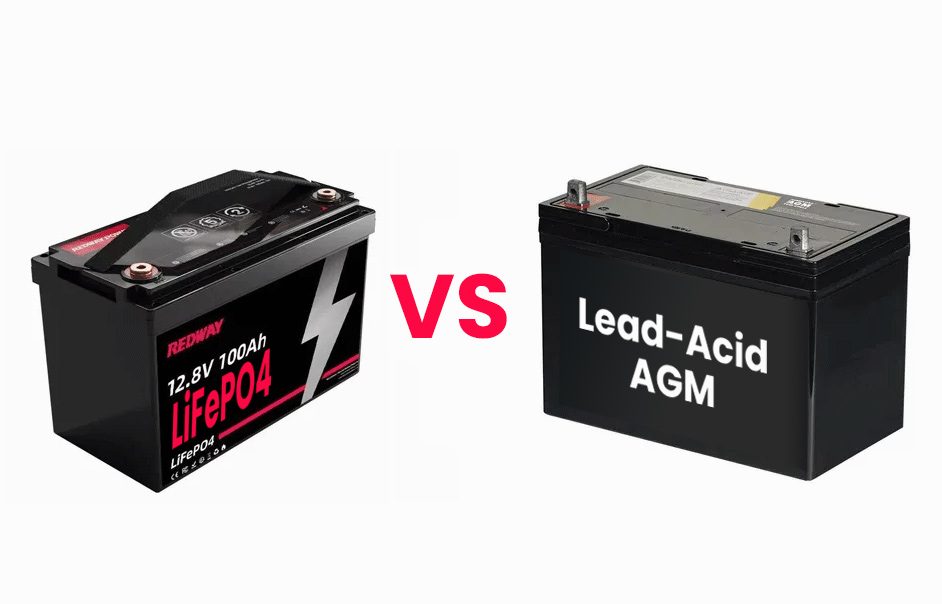
Depth of discharge capabilities differ substantially between technologies. Lead-acid batteries generally shouldn’t discharge below 50% to prevent degradation, while LiFePO4 batteries safely operate at 80-90% DoD without lifespan impact. This distinction means a 100Ah LiFePO4 battery provides nearly twice the usable capacity of an equivalent lead-acid battery.
Self-discharge rates favor LiFePO4 batteries in standby applications where batteries must remain ready for emergency use. Lead-acid batteries typically self-discharge at approximately 30% monthly, requiring frequent maintenance charging. LiFePO4 batteries self-discharge at just 2% monthly, maintaining readiness over extended periods without intervention.
Weight and space efficiency provide additional advantages in constrained applications. LiFePO4 batteries weigh approximately 70% less than equivalent lead-acid batteries while occupying about 50% less space for identical usable capacity. This efficiency proves particularly valuable in mobile systems where weight and space limitations exist.
ROI Analysis and Cost Benefits
While LiFePO4 batteries require higher initial investment than lead-acid alternatives, comprehensive ROI analysis demonstrates superior long-term economics across diverse applications.
A 10-year total cost of ownership analysis for a 10kWh solar storage system reveals striking differences between technologies:
For AGM lead-acid batteries, initial purchase costs around $2,800, but requires four replacements over a decade, adding $11,200 in replacement costs. Energy losses ($2,340) and maintenance expenses ($600) bring total 10-year ownership costs to $16,940.
For LiFePO4 batteries, higher initial costs ($6,500) are offset by eliminating replacement expenses, significantly reducing energy losses ($520), and eliminating maintenance costs. Total 10-year ownership with LiFePO4 technology amounts to just $7,020—representing 58% cost reduction compared to lead-acid alternatives.
Economic advantages extend beyond direct replacement and maintenance savings. LiFePO4’s higher efficiency means more stored energy remains available for use, reducing waste and improving system efficiency. This efficiency gain proves particularly valuable in off-grid applications where every stored kilowatt-hour represents a precious resource.
Implementing the maintenance practices outlined in this guide delivers measurable benefits: extended cycle life beyond 4,000 cycles, maintained 90% charging efficiency, and reliability in critical applications where power interruptions aren’t acceptable. Regular voltage monitoring, proper charging profiles, and temperature management represent the three pillars of effective LiFePO4 maintenance that protect your investment.
Whether you’re maintaining batteries for solar storage, backup power, or mobile applications, these evidence-based maintenance protocols ensure your LiFePO4 batteries deliver their full performance potential for years to come. For personalized maintenance recommendations specific to your application, our battery specialists are available to provide tailored guidance that addresses your unique operational requirements.